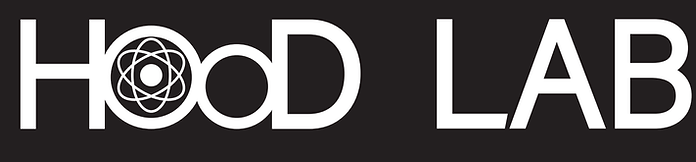
Ultracold Molecule and Quantum Optics Lab @ Purdue
RESEARCH
Our experimental goal is obtain complete quantum control of single molecules. The molecules are highly isolated from their environment and noise and model an ideal quantum system. Our bottom-up approach is to then use these molecules to build large many-body quantum systems that will give us insight into important problems in chemistry, materials, and condensed-matter physics. Our lab takes two different approaches to controlling molecules:
Laser Assembly of Single Ultracold LiCs Molecules

A strongly interacting quantum many-body system is at the heart of many of the fundamental
questions in science, ranging from material and chemical properties to black holes and lattice gauge
theory. A major goal of quantum science is to build synthetic quantum platforms that can simulate
these systems at length and time scales that can be probed with complete quantum detail.
Another major challenge is to develop new paradigms for quantum many-body systems, which
will bring new types of interactions and levels of control and coherence to bear. For example, high-
temperature superconductivity and topological phases both require long-range interaction, which
is difficult to achieve in a quantum system without becoming sensitive to environmental noise.
Polar molecules have strong anisotropic and long-range interactions via the permanent electric dipole moment. These interactions take place in rotational states of the electronic ground state. The interactions are several orders of magnitude larger than those from magnetic dipoles, and the rotational states are separated by microwave frequencies, allowing precise control and many available quantum states

Quantum Optics with Single Organic Molecules
Coherent dipole-dipole interactions are the basis for many quantum technologies. Certain polycyclic aromatic hydrocarbons are ideal platforms for quantum optics due to their lifetime limited linewidths at cryogenic temperatures and high emission onto the zero-phonon line. While organic molecules can be spaced nanometrically in a host matrix, entanglement also requires nearly resonant electronic transitions, which is hampered in the solid state by differences in local environments that
detune emitters from each other. Current methods to frequency tune solid-state emitters, such as strain and DC electric fields, are not scalable to more than two emitters. We investigate the use of a light-induced frequency tuning to generate entanglement between dibenzoterrylene molecules, with the promise of scaling to many-body interactions for quantum technologies and fundamental physics.
Recent paper: 2308.08037.pdf (arxiv.org)
